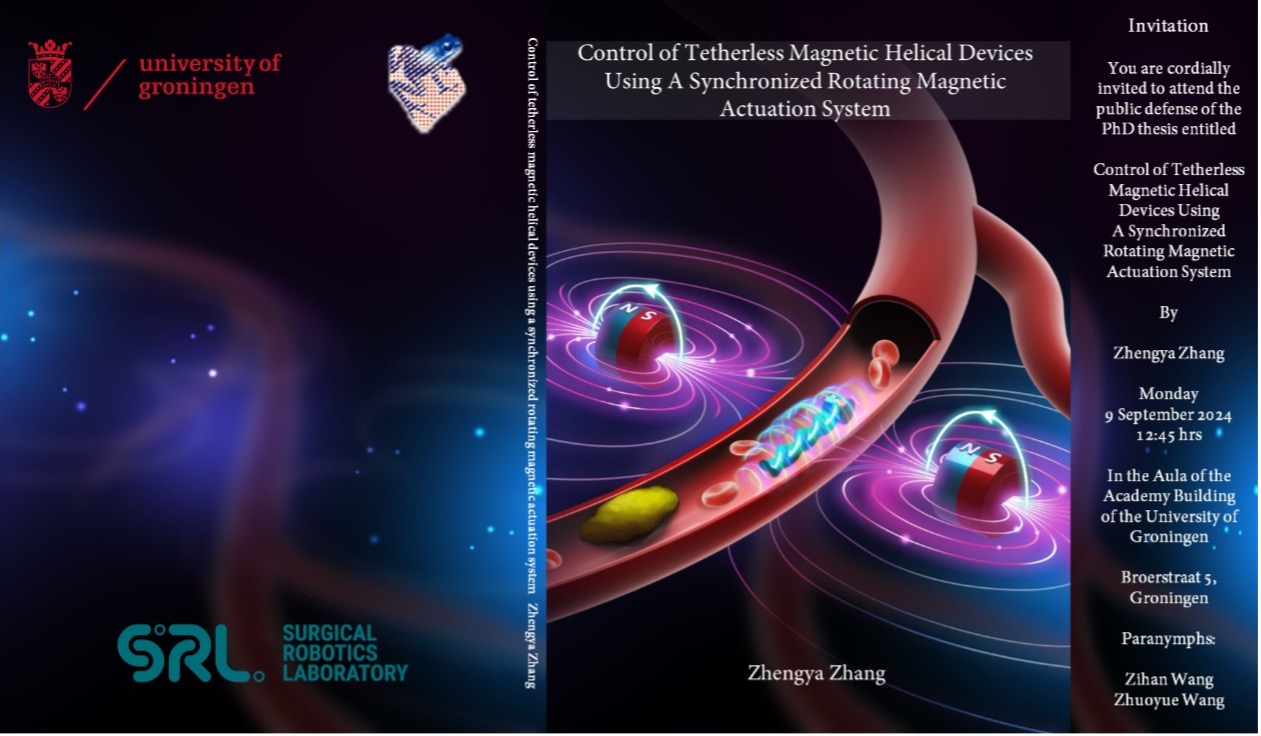
PhD Defense – Zhengya Zhang
SRL doctoral candidate defended his thesis.
The defense of the doctoral thesis titled “Control of Tetherless Magnetic Devices Using a Synchronized Rotating Magnetic Actuation System” by Zhengya Zhang was held on Monday, 9th of September 2024, at 12:4hours in the Aula of the Academy Building at the University of Groningen. The doctoral thesis is available here.
Thesis summary:
Tetherless magnetic helical devices (TMHDs), including helix-, screw-, and twist- shaped variants, exhibit considerable potential in biomedical applications, particularly for their capacity to be remotely controlled to navigate deep tissues within the human body. The primary aim of the thesis is to achieve stable and effective navigation of TMHDs in physiological environments, thereby laying the groundwork for their integration into biomedical applications such as targeted drug delivery and material removal. Chapter 1 provides a comprehensive overview of various aspects related to TMHDs, encompassing their diverse variants, magnetic actuation systems, localization sensing technologies, and their extensive applications in the biomedical field. This overview serves as a valuable reference for the development of permanent magnet-based robotic systems in Chapter 2, and the design and control of TMHDs in Chapters 3 and 4.
The magnetic field produced by permanent magnet-based robotic systems is a viable option as an external stimulus to enable the motion of a TMHD in physiological environments. Chapter 2 provides a permanent magnet-based robotic system with an open configuration using two synchronized rotating permanent magnets to generate time-varying rotating magnetic fields. These fields are used to apply torque on a TMHD in low Reynolds-number flow regimes. The configuration of the system is vertically symmetric, allowing permanent magnets to exert gradient-free space within the center of the workspace. We derive the configuration-to-pose kinematics and the pose-to-field mapping of the system. Such derivation is the basis for realizing the motion control of TMHDs in three-dimensional space. The kinematic system holds one translational degree of freedom (DOF) and three rotational DOFs, allowing it to control the pose of actuator magnets with four DOFs. The nonlinear inverse kinematic problem is solved using an optimization algorithm. The experimental results of this level of control demonstrate that the mean absolute error and the maximum tracking error of three-dimensional motion control are 1.18 mm and 2.64 mm, respectively. To attain the desired swimming behaviors of TMHDs in physiological environments, it is imperative to investigate the factors that impact their swimming behaviors in those specific conditions.
To attain the desired swimming behaviors of TMHDs in physiological environments, it is imperative to investigate the factors that impact their swimming behaviors in those specific conditions. Chapter 3 presents one variant of TMHD, called tetherless screw-shaped magnetic devices (TSMDs), which is characterized by the closed-loop behavior of an agar gel tissue phantom using our permanent magnet-based robotic system. The proposed closed-loop control strategy capitalizes on an analytical calculation of the swimming speed of the TSMD in viscoelastic fluids and the magnetic point-dipole approximation of magnetic fields. The analytical solution is based on the Stokes/Oldroyd-B equations, and its predictions are compared to experimental results at different actuation frequencies of the TSMD. Our measurements match the theoretical prediction of the analytical model before the step-out frequency of the screw, owing to the linearity of the analytical model. We demonstrate open-loop control in two-dimensional space, and point-to-point closed-loop motion control of the TSMD (length and diameter of 6 mm and 2 mm, respectively) with a maximum positioning error of 1.8 mm.
Chapter 4 presents one variant of TMHD, called tetherless twist-shaped magnetic devices (TTMDs), which is examined with an analysis of influential factors such as TTMD geometry parameters (Number of Starts, Radius, Pitch, and Amplitude) and actuation frequency on TTMD swimming speed in viscoelastic fluids. Experiments are performed in an agar gel phantom under the actuation of a synchronized rotating magnetic field. The swimming speed of each type of TTMD obtained from experiments was compared with that predicted by an existing swimming speed prediction model under a specific condition. Through this comparison, we discover the model’s effective prediction capability for TTMD swimming speed at low actuation frequencies (below the step-out frequency), yet note a decrease in accuracy at high actuation frequencies (near or above the step-out frequency). The swimming speed prediction model is then applied in the context of TTMD motion control experiments, revealing its suitability for TTMD motion control, particularly in predicting TTMD swimming speed in viscoelastic fluids at low actuation frequencies (below the step-out frequency). This capability contributes to time savings in computing TTMD position information.
Chapter 5 outlines the primary findings of this thesis, along with future directions. The present work establishes the groundwork for the subsequent stages in the application of TMHDs in biomedical engineering.
Promotor
- Prof. Dr. S. Misra (University of Twente/University Medical Center Groningen, The Netherlands)
Supervisor
- Dr. I. S. M. Khalil (University of Twente, The Netherlands)
Assessment Committee
- Prof. Dr. M. Hong (Xiamen University)
- Prof. Dr. W. Xue (Wenzhou University)
- Dr. P. K. Sharma (University of Groningen and University Medical Center Groningen)
Opposition Committee
- Prof. Dr. R. Schirhagl (University of Groningen and University Medical Center Groningen)
- Prof. Dr. F. Li (Wenzhou University)
- Dr. B. Lin (Xi’an Jiaotong-Liverpool University)